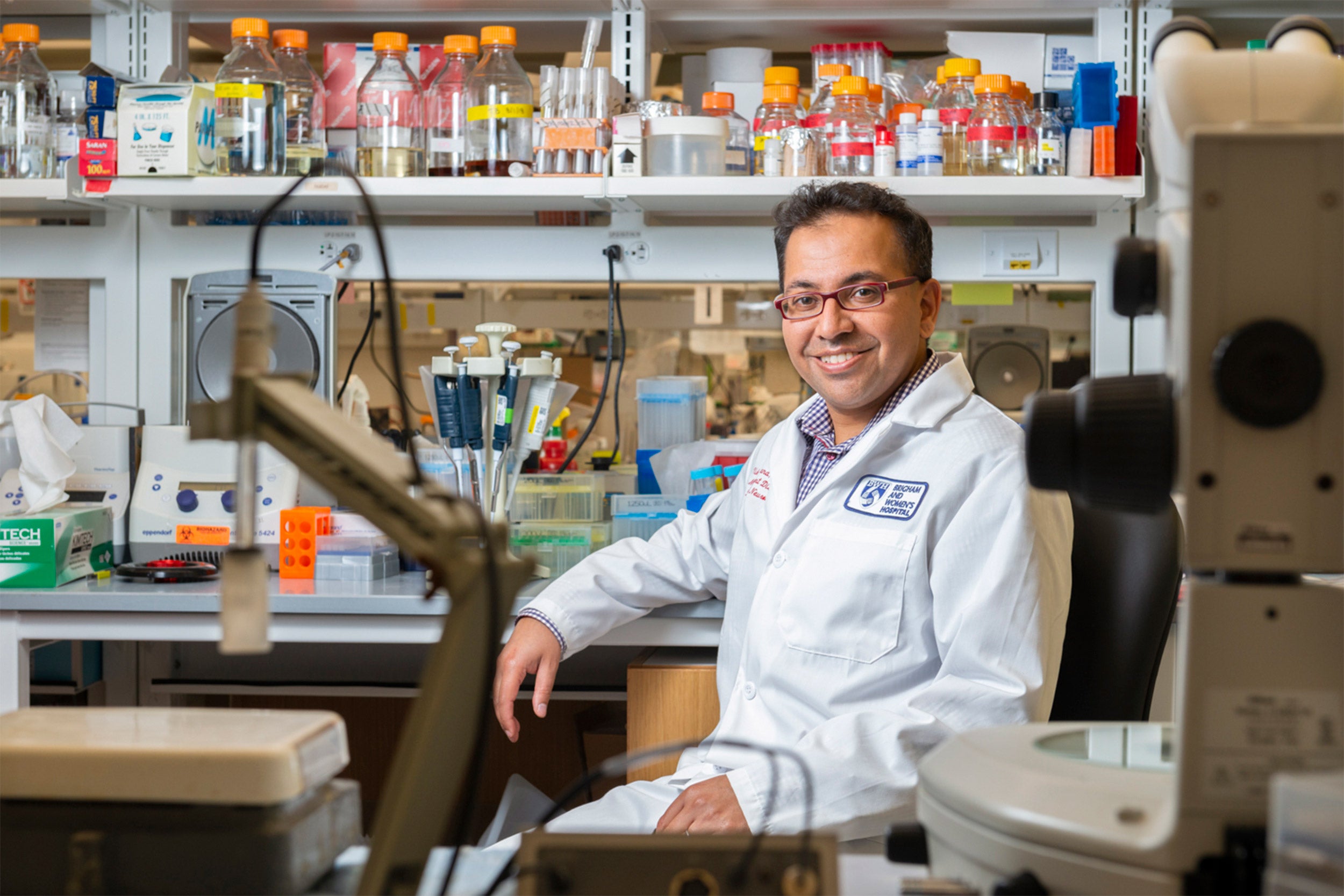
Vikram Khurana said researchers are looking at the first therapeutic trials for Parkinson’s targeting specific mutations.
Courtesy of Anna Olivella and the Harvard Brain Science Initiative
Health
Demystifying Parkinson’s
Researcher details new findings as momentum builds behind treatment efforts: ‘Every problem looks intractable until it’s solved’
A team led by researchers at Brigham and Women’s Hospital and Harvard Medical School has taken a step toward solving a central mystery of Parkinson’s disease: What is the normal function of the protein whose misfolding causes the condition’s characteristic clumps in neurons?
In an interview with the Gazette, the study’s senior author, Vikram Khurana, discussed recent advances and new, individualized approaches to Parkinson’s, as well as implications of the recent findings, which were achieved with key contributions from instructor in neurology Erinc Hallacli and postdoctoral fellow Sumaiya Nazeen. The interview has been edited for clarity and length.
Q&A
Vikram Khurana
GAZETTE: Have we been making progress toward new treatments for Parkinson’s?
KHURANA: Enormous progress is being made, but there are big challenges. One is understanding what causes the disease. We’ve made considerable progress in understanding genetic and environmental causes and understanding how those may fit together. We’re seeing the first therapeutic trials for Parkinson’s targeting specific mutations. There is general consensus that going for a subtype of Parkinson’s that we can understand molecularly will be a more fruitful approach and that a one-size-fits-all strategy may not work for Parkinson’s and other neurodegenerative diseases. Another challenge we are addressing is clinical trial design. The right treatment comes to nothing if the clinical trial is not designed well. We’re getting better at that.
GAZETTE: Alpha-synuclein is the protein whose misfolding is characteristic of Parkinson’s disease. Do we know what normal alpha-synuclein does?
KHURANA: When you knock alpha-synuclein out of a mouse, the mouse is pretty much fine, but when you look in more detail at the neurophysiology of the mice and the neurons that you make from those mice, you see defects in the way those mouse neurons are signaling to each other. Alpha-synuclein is involved in trafficking little balloon-like structures in neurons and other cells that are called vesicles. Alpha-synuclein sticks to the surface of the balloons, at a fatty layer known as a membrane. Vesicles carry around not only proteins, but, in a neuron, also carry around the key signaling molecules that transmit messages between the cells — neurotransmitters. It’s important to note that, while the field understandably became obsessed with what alpha-synuclein is doing in neurons, it exists in high abundance in other cell types: red blood cells, kidney cells.
GAZETTE: Do we know how misfolded alpha-synuclein causes disease?
KHURANA: Typically, these are proteins found in high abundance and have a property — first described for prions — where when they misfold and come into contact with normal counterparts, they trigger the misfolding of those normal proteins. This provides a mechanism through which the pathology can spread within a cell and between cells; a chain reaction.
“There is general consensus that going for a subtype of Parkinson’s that we can understand molecularly will be a more fruitful approach and that a one-size-fits-all strategy may not work for Parkinson’s …”
GAZETTE: You mentioned that alpha-synuclein is useful in transporting vesicles. In the new work you found a second function?
KHURANA: Alpha-synuclein is also abundant in the cell away from the vesicle membrane. What is it doing there? Some years ago, our lab created the first cellular “road maps” of alpha-synuclein: We used methods in molecular biology to identify genes that, when altered, influence alpha-synuclein toxicity or proteins in very close proximity to alpha-synuclein. We were expecting other proteins associated with vesicle membranes and vesicle trafficking, and we saw them. But we also saw another group of proteins that were very over-represented called RNA-binding proteins.
RNA-binding proteins regulate mRNA, which are the messenger molecules in our cells responsible for transcribing DNA into proteins. This suggested that alpha-synuclein was involved in gene regulation. I think this likely happens in many ways, but, fast-forward about five years, and in this paper we’ve discovered that the very same part of alpha-synuclein that binds to membranes — the so-called N-terminus — has a double life and interacts with cellular machinery called P-bodies, a conglomerate of RNA-binding proteins in the cell that regulate the degradation of mRNA. So there’s a dichotomy: If you’re at the membrane, you’re not going to be in the P-body; if you’re in the P-body, you’re not at the membrane. And if you disrupt the membrane, you can alter the regulation of genes.
GAZETTE: So is it possible that synuclein is disrupting the P-bodies and slowing down the degradation of mRNAs?
KHURANA: That’s what we found. We show this in a few ways in the paper. For example, these days we can actually reprogram the cells of our patients into human embryonic stem cell-like cells called iPSC cells. We did this for patients born with two extra copies of alpha-synuclein. In total, they have four copies of protein instead of just two. Those patients get a very aggressive form of Parkinson’s disease and dementia. We made these cells, converted them into neurons, and asked, “With pathologic levels of alpha-synuclein, what happens to P-bodies and what happens to the stability of mRNA?” We saw a signal which showed that the P-body complex — this mRNA-degradation complex — was falling apart; it wasn’t forming the right structure. And we hypothesize that that’s because alpha-synuclein is interacting so strongly when it’s at a pathologic level with some of these P-body components that those P-body components can no longer find their physiologic partners. In addition, as you might expect, the mRNA was indeed abnormally stabilized because the P-body is now not able to function appropriately in mRNA degradation.
GAZETTE: Why is knowing this potentially important?
KHURANA: Putting aside Parkinson’s disease for a moment, gene regulation is very important and we think small chameleon proteins like alpha-synuclein — which we call intrinsically disordered — are ideal sensors, relaying biology in distant parts of a cell and leading to responses in gene regulation. The dichotomous interaction I describe for alpha-synuclein is a very elegant way for a cell to respond to alterations of vesicle trafficking. So that’s the biology. But this might also be really important for Parkinson’s and other synucleinopathies, too.
First, we discovered patients with P-body protein mutations may be at higher risk of Parkinson’s disease. This immediately suggests that among P-body proteins there may be clues for how the disease arises in some patients and what it might take to treat those patients. And on the therapeutic side, our data introduces a level of unanticipated nuance. We know if you inherit extra copies of the alpha-synuclein gene, you end up with early aggressive Parkinson’s and dementia. If you have common genetic variants that give you 15 or 20 percent more alpha-synuclein, you’re also at higher risk of getting Parkinson’s disease. So there’s a solution circulating out there: “Well, too much of these proteins is clearly a bad thing, genetics suggest that. So why don’t we develop therapies to lower the concentration of this protein?” It’s a simple idea worthy of testing. But over the last couple of decades in the Alzheimer’s space we haven’t seen that lowering the level of aggregation-prone proteins has yielded convincing dividends for patients. That might change. But regardless, if you target a protein like alpha-synuclein, it’s important to know what that protein does. If you do not know what your target protein does, you lower its levels at your peril. You’re at risk of not knowing how to dose your therapy — you are flying blind and might end up creating more of a problem than you started with. Moreover, if a protein like alpha-synuclein starts to aggregate, that aggregation could take the normal soluble form of the protein away from where it should be. By the time a protein is aggregating in a neuron, knocking that protein down may actually be counterproductive. Tuning, rather than lowering, alpha-synuclein back to a more physiologic state may be a better strategy. But you can’t define a physiologic state if you don’t have a function. Our work deepens our understanding of that function.
“Every problem looks intractable until it’s solved, and sometimes progress comes very abruptly. I hope this proves true soon for neurodegeneration.”
GAZETTE: Neurodegenerative diseases seem particularly difficult nuts to crack scientifically — why is that?
KHURANA: Every problem looks intractable until it’s solved, and sometimes progress comes very abruptly. I hope this proves true soon for neurodegeneration. From where we stand right now, one major issue is the chronicity of these diseases and the other is the heterogeneity of the disease. They are interrelated.
First, let’s take chronicity. Diseases like Parkinson’s play out over many years, probably decades. Vulnerabilities to Parkinson’s pathology can be identified by labs like ours even in fetal maturity neurons we make from patient stem cells. Designing a clinical trial for a chronic disease is very challenging: In six months or a year, we have to see a signal that convinces us about a treatment for a chronic disease. On the one hand, recruiting patients who are early in their disease course may be ideal for disease-modifying treatments. On the other hand, some patients may be progressing fast and others very slow. A mix of such patients may create too much noise to observe any therapeutic efficacy, even with a drug that “works.”
And of course, even within the brain of a single patient, it’s conceivable that different brain regions will need different treatments. For example, Parkinson’s patients typically come to our clinic when they have a movement disorder, but in the latter half of the disease, nonmotor symptoms like dementia and psychosis can become much more prominent and debilitating. At the time of presentation, we may need to be in prevention mode for the dementia, but in treatment mode for the motor disease, and those therapies might be quite different. In time, perhaps we will need combinations of strategies.
And then there is heterogeneity. Not only are patients progressing at different rates when we first diagnose them, but Parkinson’s disease may be triggered in different ways in different patients. At the end stage, the disease may look quite similar but the pathways to get there may be different. These different paths may need different strategies.